AI-Powered Patent Review and Analysis - Streamline Your Patent Process with patentreviewpro.com (Get started for free)
Recent Advances in Aluminothermic Welding for Rail Track Maintenance A 2024 Update
Recent Advances in Aluminothermic Welding for Rail Track Maintenance A 2024 Update - Semiautomated Welding Systems Boost Track Maintenance Efficiency
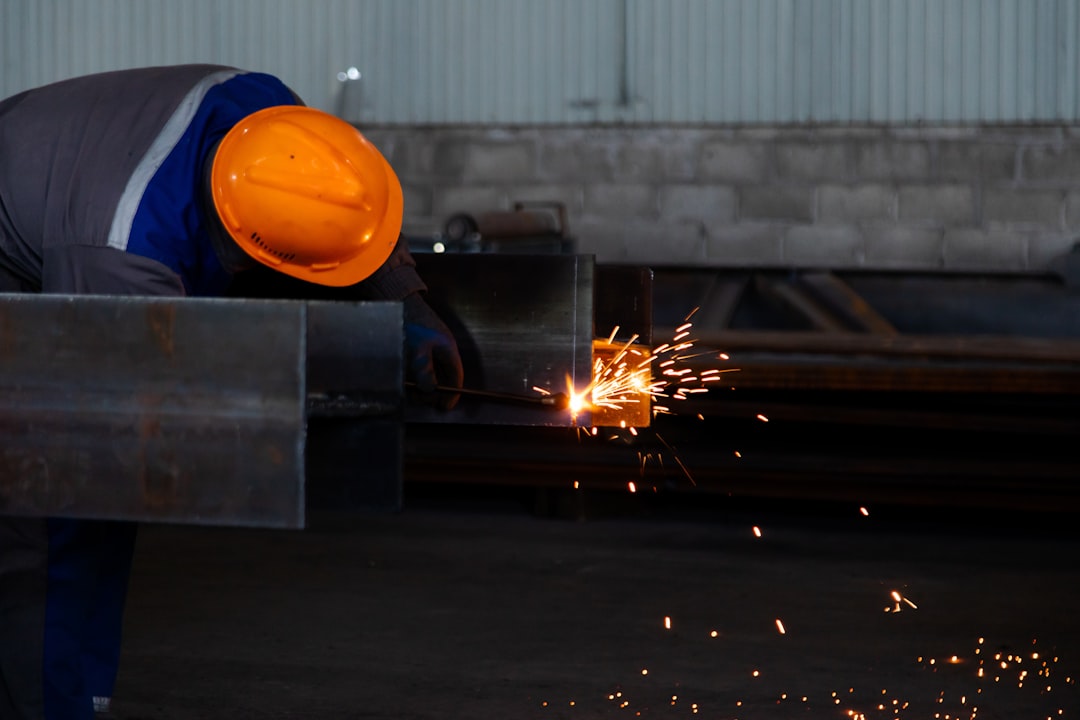
Semiautomated welding systems are transforming rail track maintenance by significantly improving efficiency while keeping costs in check. These systems particularly benefit aluminothermic welding, making it a more robust technique, especially for tracks carrying heavy loads. The use of digital tools like simulation and rapid prototyping is accelerating the refinement of aluminothermic welding methods. The ability to weld rails on-site, without needing to disassemble the track, makes this approach exceptionally valuable. Aluminothermic welding's adaptability to different working conditions has solidified its position as a favored technique within the industry. Ongoing research is critical to fully understand the thermal and mechanical complexities of the welding process, which will ultimately lead to better weld quality and reliability. Nonetheless, the potential for certain types of weld failures serves as a reminder of the importance of ongoing process improvements and careful monitoring to avoid these issues.
Semiautomated systems are emerging as a game-changer for aluminothermic welding in rail track maintenance, particularly due to their potential to slash repair times. Reports suggest these systems can cut repair time by as much as 50%, getting tracks back into service much quicker. This speed increase comes from sophisticated sensors and computer-controlled algorithms that precisely guide the welding process. This degree of control lessens the chances of human mistakes that frequently plague manual welding.
The flexibility of these systems has also seen improvements, with current iterations showing the ability to handle various rail profiles. This is crucial because it means they can be readily applied across a wider variety of track designs. While this is certainly an advantage, the question remains whether this flexibility translates to consistency in different conditions.
An intriguing aspect is how the heat-affected zone (HAZ) in semiautomated systems seems to be narrower compared to conventional methods. This leads to less distortion and potentially stronger joints, especially critical for heavy rail traffic. While this is encouraging, I'm curious to see more evidence demonstrating this claim across different weld types.
Integrating machine learning is a natural progression in this area. The real-time analysis it provides could enable us to move toward more predictive maintenance schemes, which is vital to prevent unforeseen track failures. But the long-term reliability of such systems in diverse conditions still requires further investigation.
Further, advanced cooling technologies integrated into these systems seem to expedite the cooling process after welding. This has the potential to refine the mechanical properties of the resulting weld. However, I'd like to see comprehensive analysis of the impacts on weld microstructure and tensile strength.
The data-logging and diagnostics built into these systems is an additional positive aspect. It's encouraging to see the potential for better monitoring of the weld integrity over time, but the robustness of these diagnostic capabilities needs to be scrutinized in field trials.
Besides the efficiency gains, semiautomated welding reduces the required training time for operators. While it's great to see operator training becoming more streamlined, it's important to assess whether this shortened timeframe affects the quality of operator competency.
The portability of these new systems holds great promise for addressing maintenance in remote or less-accessible locations. This could address a critical logistical challenge in the rail sector, but it remains to be seen how these systems function in harsh environmental conditions and with challenging terrain.
Finally, the enhanced precision of these systems not only leads to stronger welds but also promotes more consistent weld profiles. This aspect could definitely contribute to smoother train rides, though further study of the correlation between specific weld profiles and ride quality is needed.
Recent Advances in Aluminothermic Welding for Rail Track Maintenance A 2024 Update - New Fatigue Testing Methods for Aluminothermic Rail Welds
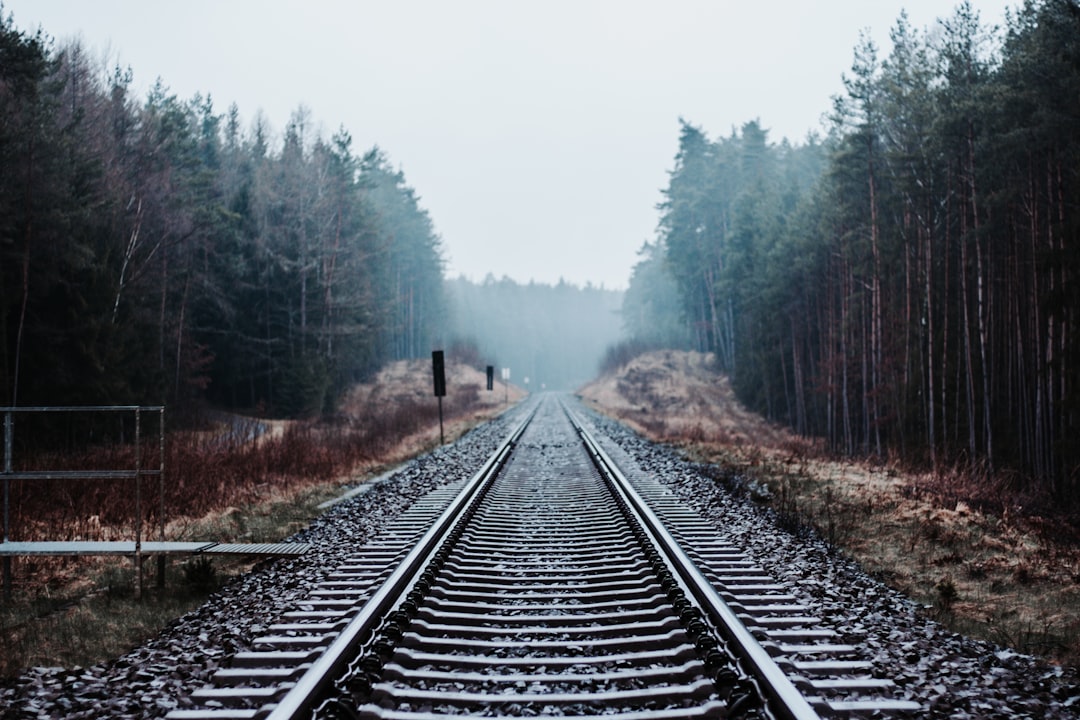
Aluminothermic welding, while a long-standing and simple method for rail track repairs, is facing increased scrutiny due to a rise in premature weld failures, particularly under the stress of heavy axle loads. This issue has become more pronounced in newer welds, whether on straight or curved track sections. A major failure mode identified in these welds is the Horizontal Split Web (HSW), where fatigue cracks often originate from surface or subsurface defects within the weld collar.
To address these concerns, researchers have begun using sophisticated multiaxial fatigue analysis, incorporating dynamic assessment techniques to understand the complex stresses experienced by welds under heavy traffic. This approach seeks to evaluate the weld performance more accurately than previous methods.
However, a key challenge remains in the inconsistent quality of aluminothermic welds due to the reliance on operator skill and experience. This process variability can lead to casting defects and variations in microstructure and mechanical properties, influencing the weld's resistance to fatigue.
With rail traffic and axle loads increasing, ensuring the long-term integrity of these welds is becoming ever more critical. Consequently, the advancement of fatigue testing methods holds significant importance for predicting weld reliability and enhancing the durability of rail track systems. Improving our analytical tools to better assess the risks of failure under operating conditions is essential for maintaining rail safety and minimizing future disruptions.
Aluminothermic welding (ATW), a long-standing method for rail repair, is seeing renewed scrutiny due to an uptick in premature weld failures, particularly in continuously welded rail (CWR) lines under heavy traffic. This has prompted a closer look at the fatigue characteristics of these welds, leading to the development of new testing methods that aim to better predict their performance in real-world conditions.
These new approaches emphasize the use of high- and low-cycle fatigue tests to mimic the repetitive stresses experienced by rails. Instead of relying on older, more general tests, researchers are creating test specimens that more accurately reflect the actual geometry of aluminothermic welds, thus improving the relevance of the test data. One intriguing observation is how temperature fluctuations, a natural part of service, can significantly impact fatigue life. This has led to the integration of temperature control into fatigue testing to more closely mirror real-world scenarios, potentially leading to more realistic predictions.
Connecting the mechanical properties of the weld and its heat-affected zone to fatigue performance is a key focus of these new methods. This is a crucial step in developing better predictive models for rail infrastructure lifespan. Furthermore, these tests are moving beyond simple static load simulations to replicate the dynamic stresses experienced under actual train passage. By mimicking these conditions, researchers can get a better understanding of how specific failure modes emerge under such dynamic loading.
Real-time non-destructive testing (NDT) integrated with the fatigue tests is also a promising development. This enables a constant assessment of microstructural changes without the need for destructive testing, allowing for a more continuous and informative view of how the weld reacts to cyclic stresses. Additionally, there's a growing effort to understand how alloying elements used in filler materials influence fatigue resistance. This opens up the possibility of strategically adjusting weld composition to enhance fatigue life.
The weld's interaction with different rail substrates is also being incorporated into fatigue testing, highlighting the importance of this interplay in overall weld durability. With a deeper understanding of these interactions, we can potentially design weld solutions tailored to specific rail materials. These new approaches are also feeding into the development of better models for predicting fatigue limits. By incorporating historical performance data and employing statistical methods, we can develop more reliable estimates of how long welds will remain functional, aiding in infrastructure planning and maintenance schedules.
The data gathered from these improved fatigue tests is valuable in developing a closed-loop system for improving the welding process itself. The insights gained from fatigue testing can be fed back to the welders and engineers, allowing them to continuously refine their techniques and, hopefully, achieve more consistent, fatigue-resistant welds. This approach to testing, with its focus on simulating real-world conditions and providing a mechanism for process improvement, represents a significant step towards improving the reliability of aluminothermic rail welds in the face of increased operational demands.
Recent Advances in Aluminothermic Welding for Rail Track Maintenance A 2024 Update - Addressing Premature Weld Failures in High Axle Load Conditions
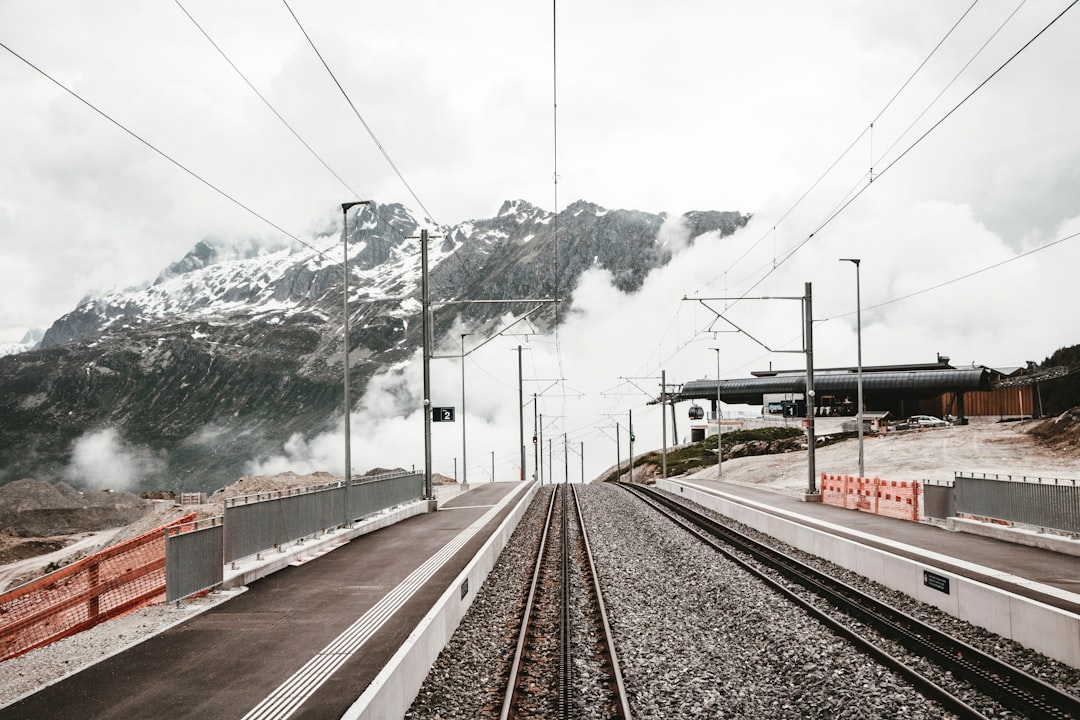
The rise in premature weld failures, particularly in high axle load conditions, has brought the limitations of aluminothermic welding into sharper focus. As the rail industry leans more heavily on continuously welded rail (CWR) systems, the need for robust weld integrity becomes crucial. Unfortunately, the increase in heavy rail traffic has led to a surge in failure modes like Horizontal Split Web (HSW), often originating from defects within the weld zone. This raises significant safety concerns. Addressing these issues has spurred the development of advanced fatigue testing methods that strive to replicate the intricate stress patterns experienced in real-world rail operation, thus allowing for a more nuanced understanding of weld performance under these harsh conditions. However, a persistent challenge remains—the inherent variability in weld quality due to operator expertise. Overcoming this will likely involve refining training programs and implementing stricter standardization measures across the welding process, ultimately aiming to elevate the overall reliability and longevity of rail track welds.
Aluminothermic welding (ATW), a foundational rail welding method, is experiencing a surge in premature weld failures, particularly under high axle load conditions on both straight and curved tracks. This issue is concerning, especially as we've moved toward continuously welded rail (CWR) systems, largely because of their superior performance and reduced maintenance compared to older methods. These high axle loads demand top-notch weld quality and material properties; failed welds can cause significant safety issues, including derailments.
The quality of aluminothermic welds, unfortunately, can vary considerably due to factors like operator skill. These inconsistencies can lead to defects like casting imperfections or variations in the weld's microstructure and mechanical properties. This means the probability of a weld failure might be higher than that of the parent rail itself in environments with high axle loads.
In response to these challenges, researchers and engineers are using Failure Mode and Effects Analysis (FMEA) to identify and address the root causes of these weld failures. However, it's a bit odd that typical track maintenance, like rail grinding, doesn't seem focused on addressing weld batter specifically. It suggests there's a gap in how we think about welding in the maintenance cycle.
A more rigorous, analytical approach to evaluating fatigue behavior in aluminothermic welds is developing, and it's promising for bolstering their reliability in harsh conditions. These failures have led to a serious reassessment of the standards for these welds within the railway industry.
Researchers are delving into the intricate details of how welds perform under dynamic loading conditions. It turns out that temperature changes during normal service can substantially impact fatigue life in the weld. This realization has pushed us to include temperature as a crucial element in our fatigue testing.
While the variability in weld quality due to operator influence continues to be a challenge, a promising development is the use of non-destructive testing (NDT) integrated with fatigue tests. This approach allows us to observe internal changes within the weld without destroying it, giving us continuous insight into how it's responding to repetitive loads.
Furthermore, the influence of different alloying elements within filler materials on fatigue performance is being explored. This suggests the exciting possibility of tailoring the weld composition to specific operating environments, enhancing its durability. Additionally, we're starting to understand the interplay between welds and different rail materials, leading us toward weld solutions that work well with each specific type of rail.
All of this is leading to the development of refined models for predicting weld fatigue limits. Using statistical methods alongside existing weld performance data, we can improve the accuracy of our predictions of how long welds will last. This has obvious implications for infrastructure planning and maintenance scheduling. This information, along with the analysis of weld failures, can be looped back into the welding process, allowing for continuous improvement of the process itself. Hopefully, this leads to better, more durable welds.
Recent Advances in Aluminothermic Welding for Rail Track Maintenance A 2024 Update - Innovations in Digital Simulation for Improved Weld Properties
Digital simulation is increasingly important in refining aluminothermic welding techniques, especially in rail track maintenance. Researchers are using computational tools to better understand the complex interplay of heat and mechanics during the welding process. This includes monitoring welding parameters in real-time and creating digital representations (digital twins) of the welding process. The aim is to improve control over the process and achieve more consistent weld quality. Software specifically designed for welding, like NovaFlow & Solid CV, is being employed to predict certain weld properties, such as the length of the weld and heat-affected zones. This ability to predict weld characteristics has the potential to help solve challenges like the large amount of weld material often used in these processes. As these innovations continue to mature, there is hope that not only will the properties of the weld be improved but that the entire welding process will become more reliable, contributing to more robust rail infrastructure. However, some questions remain regarding the long-term reliability of these digital tools in a variety of welding conditions.
Pandrol's focus on digital tools, like simulation, for improving aluminothermic welds is quite interesting. While aluminothermic welding has been around for a long time, its simplicity and on-site application still make it a valuable technique, especially for heavy haul rail tracks. The basic process, involving pouring molten metal into a mold surrounding the rail gap, hasn't changed much, but innovations like "iweld" are changing how it's done, with automation and data management boosting quality and productivity.
Researchers are increasingly using computational tools like NovaFlow and Solid CV to model the process more thoroughly. This type of modeling, though still under development, could allow us to predict weld and heat-affected zone (HAZ) lengths. And the use of digital twins, which are basically virtual representations of the weld process, could help visualize weld parameters and robot movements in real-time. This would be great for monitoring the entire welding system.
There's also a drive to find ways to reduce the amount of weld metal added, as it can lead to complications when welding heavy haul rails. A better understanding of the thermal and mechanical aspects of the welding process could help here, particularly examining how phase changes within the materials affect the process.
However, there's more to these new approaches than just improving the overall process. There seems to be a focus on enhancing the quality and predictability of the welds, making them more consistent and resilient. This is crucial given the increase in failures we're seeing, especially with heavy traffic and the prevalence of continuously welded rail. It's certainly promising that these new digital tools have the potential to provide this greater control over the process, leading to more reliable track systems overall. It will be fascinating to see how these changes evolve, and whether they can indeed create a notable difference in the quality of the weld joints over time. I remain curious about whether we'll see a marked reduction in those weld failures we've seen recently.
It also seems interesting that these new tools are helping to understand how specific welding parameters affect the microstructure of the weld. This type of insight could be important for tailoring welds to different types of rail material or to specific loading conditions. There's also a push to look at the whole system with multi-scale simulations, bringing together the microscopic and macroscopic views of the weld.
And of course, with all these tools creating lots of data, there's also an opportunity to learn more about how the welds fail. With improved models for predicting failure modes, it might be possible to refine the welding process to prevent certain failures from happening in the first place. I do wonder if all these potential improvements will really lead to the more consistent welds we're hoping for. But the initial results are encouraging. It seems that digital simulation and modeling could be a valuable tool for solving long-standing challenges in this area, moving toward more durable and reliable rail systems for decades to come.
Recent Advances in Aluminothermic Welding for Rail Track Maintenance A 2024 Update - Advanced Numerical Modeling of Aluminothermic Cooling Processes
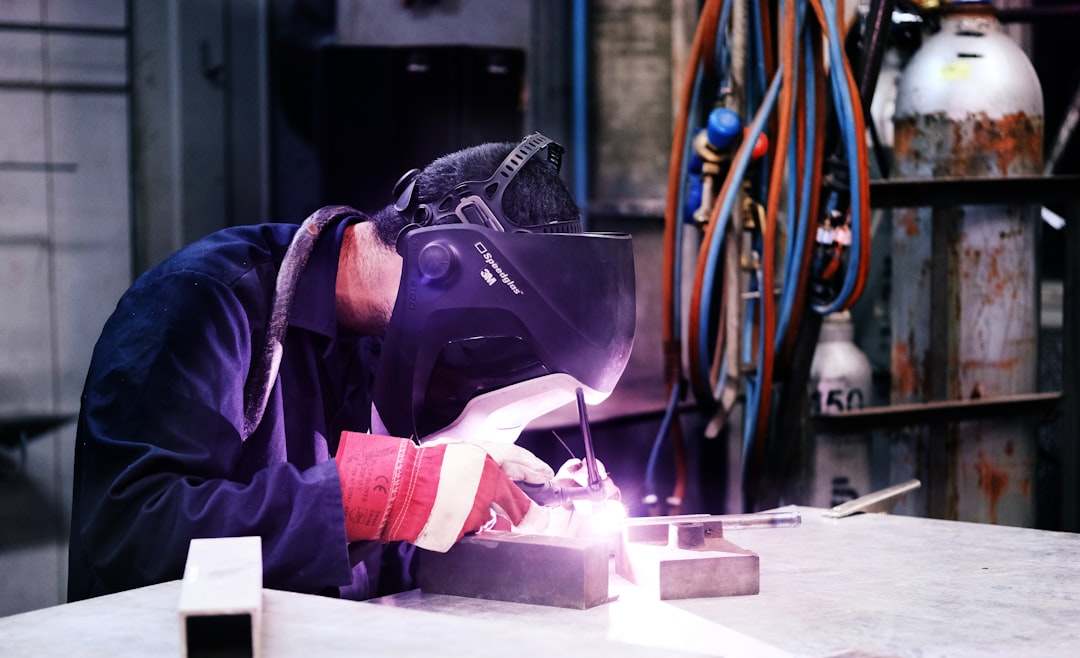
Advanced numerical modeling has emerged as a key area for improving aluminothermic cooling processes in rail track welding. These models, often built using software like OpenFOAM, are designed to simulate heat transfer and the solidification that occurs during the cooling stage after the weld is made. A significant focus has been on simulating rapid welding techniques that don't require preheating, making the modeling process simpler. This simplification allows for a more detailed examination of the thermal and mechanical behaviors that occur during welding.
These models provide valuable insights into how temperature spreads throughout the cooling process, which is crucial for understanding potential defects that can occur in the weld. These insights are vital for ongoing efforts to refine aluminothermic welding practices and ensure the welds' long-term integrity. The potential for these models to predict defects is particularly important, as defects can compromise the weld's structural integrity. While the use of computational models like OpenFOAM shows promise, their full potential to improve weld quality and consistency in real-world rail maintenance remains to be fully realized. Further research and development in this area may help optimize welding parameters to create more reliable and robust welds.
The cooling phase in aluminothermic welding is increasingly being scrutinized, with numerical modeling playing a pivotal role in understanding its intricacies. Researchers have discovered that the speed at which the weld cools significantly impacts the grain size in the heat-affected zone. Interestingly, finer grain structures often translate to improved mechanical properties, which are essential when dealing with the heavy loads placed on rail tracks.
Furthermore, it seems that altering the shape of the cooling mold can lead to more efficient heat transfer, potentially reducing the overall cooling time. Such optimizations are not merely academic pursuits; they can translate into tangible gains, like faster repair times and improved operational efficiency.
The initial temperature distribution within the weld also seems to be a major factor in shaping the resulting microstructure. For example, steeper temperature gradients might lead to more refined structures, enhancing a weld's fatigue resistance under the rigors of heavy train traffic.
Numerical simulations have proven valuable in mapping the stress distribution within the weld itself. This capability helps engineers pinpoint potential failure locations and tweak the welding parameters to mitigate localized stress concentrations.
Going further, researchers are leveraging advanced modeling to forecast crack formation and propagation under cyclic loading. This ability to predict how cracks might evolve could eventually lead to proactive measures during the welding process to minimize failure risks.
Exploring various alloy compositions within the welding materials has also yielded interesting results. Specific alloy combinations appear to influence the cooling behavior and, ultimately, the weld's integrity. The challenge lies in finding alloy mixes that enhance ductility while maintaining the required strength for rail tracks.
Numerical modeling has also illuminated innovative approaches to controlling the weld's temperature during the cooling process. Active temperature control, facilitated by novel techniques, enables engineers to maintain the weld within precise temperature ranges throughout the cooling phase, contributing to better quality and consistency.
Bridging the gap between the microscopic world of metallurgical changes and the macroscopic performance of the weld is a major focus of researchers. Multi-scale modeling offers a more holistic view, potentially leading to better welding methods and improved weld durability.
The speed at which the welding process is executed also seems to have a substantial effect on the weld's structure and its ultimate properties. Finding the optimal welding speed could minimize defect formation and enhance the desired characteristics of the weld.
The combination of machine learning and numerical modeling is leading towards more sophisticated predictive maintenance tools. By analyzing data and implementing machine learning algorithms, we might be able to anticipate potential failures in real-time. This would permit engineers to take preemptive actions to extend the service life of welded rail joints, particularly in environments with heavy axle loads and the potential for frequent cycling.
While these advancements are promising, there are still many aspects of the aluminothermic welding process that require further investigation. Nevertheless, these research avenues show how numerical modeling is transforming our understanding of this vital technology, driving innovation and paving the way for more resilient and durable rail track systems.
Recent Advances in Aluminothermic Welding for Rail Track Maintenance A 2024 Update - Implementation of Phased Array Inspection for Early Flaw Detection
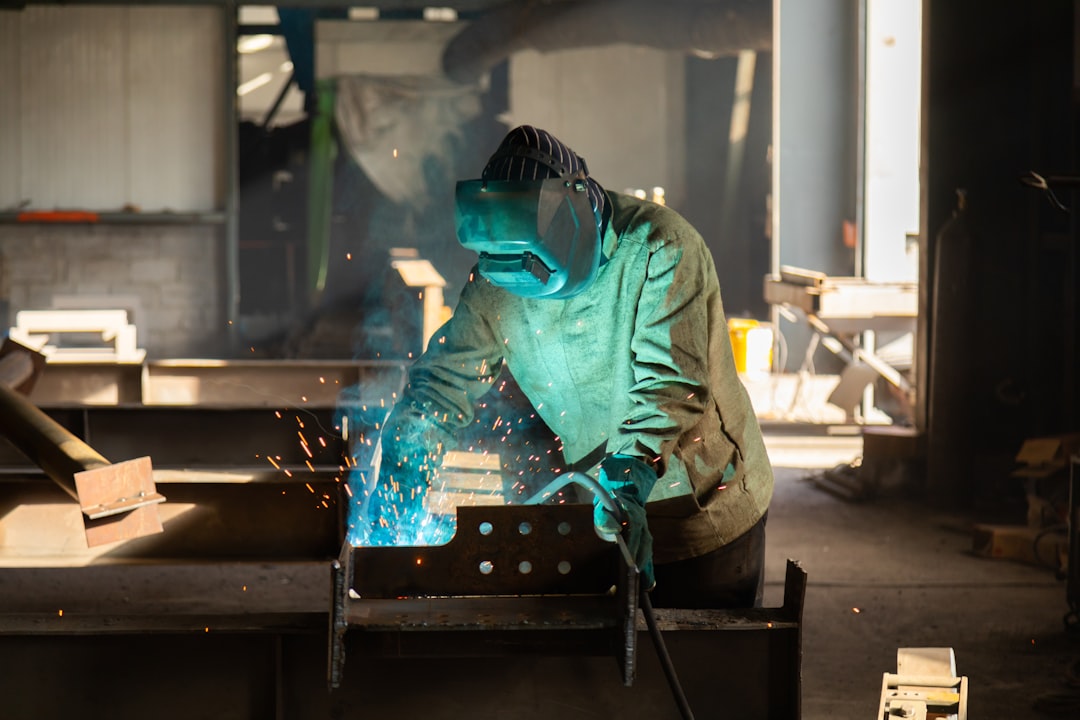
Phased array ultrasonic testing (PAUT) is emerging as a significant tool for early flaw detection in rail track maintenance, particularly within aluminothermic welds. Its enhanced sensitivity, especially in dealing with complex materials like austenitic steel, makes it a promising technique for detecting flaws that might otherwise go unnoticed. This is particularly useful given the challenges these materials present, including anisotropy and inhomogeneity.
One interesting development is the use of linear phased array inspection to replicate different flaw orientations, potentially improving the accuracy of defect identification within the rail tracks. Additionally, the use of artificial intelligence, specifically convolutional neural networks, for automated ultrasonic signal analysis has shown accuracy comparable to human inspectors. This approach could be a game-changer for inspection efficiency and consistency.
Another promising advancement is the refinement of probe positioning techniques during PAUT inspections. This aspect is crucial for ensuring that the inspection process is thorough and accurate, especially in complex track configurations.
The increased use of PAUT signifies a movement towards more sophisticated and robust inspection techniques in rail maintenance. This trend is critical for ensuring the long-term integrity and safety of rail infrastructure. The continuing development and implementation of PAUT will likely be central to addressing the growing concerns about weld reliability and maintaining the operational integrity of rail networks. However, it remains to be seen how effective these methods will be in diverse rail track environments.
Phased array ultrasonic testing (PAUT) has emerged as a promising technique for detecting flaws in aluminothermic welds, particularly in austenitic steels, which can be challenging due to their inherent anisotropic and inhomogeneous nature. The ability to replicate various orientation effects using linear phased array probes on rail tracks suggests an improvement over conventional methods in finding flaws. Interestingly, convolutional neural networks have been employed to automate the analysis of ultrasonic signals with results comparable to human experts, which is crucial in streamlining inspection processes.
The importance of detecting rail flaws, especially for heavy haul operations where the consequences of failure are heightened, has been highlighted in the research. Existing ultrasonic inspection approaches face some drawbacks, including limited coverage and the difficulty in adjusting inspection angles. High-speed phased array systems are being developed to overcome these limitations.
There are hints that acoustic emission techniques might become a more prominent method for detecting rail track faults, although it's still in its early stages of investigation. These advances in phased array technology have led to more refined techniques for positioning probes during inspections, which can contribute to a more thorough evaluation.
Comparative studies of immersion testing results and scans from rail search units have provided a good foundation to assess where modeling and inspection methodologies can be improved. The current efforts in PAUT research appear aimed at refining industry standards and procedures for rail maintenance, which is promising but may face challenges given the complexity and diversity of weld types used in rail tracks. Overall, it seems phased array ultrasonic testing represents a significant step toward creating more reliable and comprehensive inspection methods for rail infrastructure, a crucial aspect for enhancing the safety and performance of railway systems. It remains to be seen whether the benefits of phased array fully translate across all rail track environments and different types of weld geometries. However, the early results and ongoing developments point towards an exciting future for phased array technology in rail maintenance.
AI-Powered Patent Review and Analysis - Streamline Your Patent Process with patentreviewpro.com (Get started for free)
More Posts from patentreviewpro.com: